In 2018 Arthur Ashkin won the Nobel Prize in Physics “for the optical tweezers and their application to biological systems”. In essence, optical tweezers are highly focused laser beams and they could serve as an incredibly sensitive set of tweezers to manipulate and study single cells and biomolecules. Particularly, they are able to apply forces in the pN-range and to measure displacements in the nm-range of objects ranging in size from 10 nm to over 100 μm. Our group are focusing on developing various optical tweezer techniques and exploring their applications at single cellular and single molecular level. The major techniques that are under development include optical tweezers for living animals and laser tweezers Raman spectroscopy. Read More
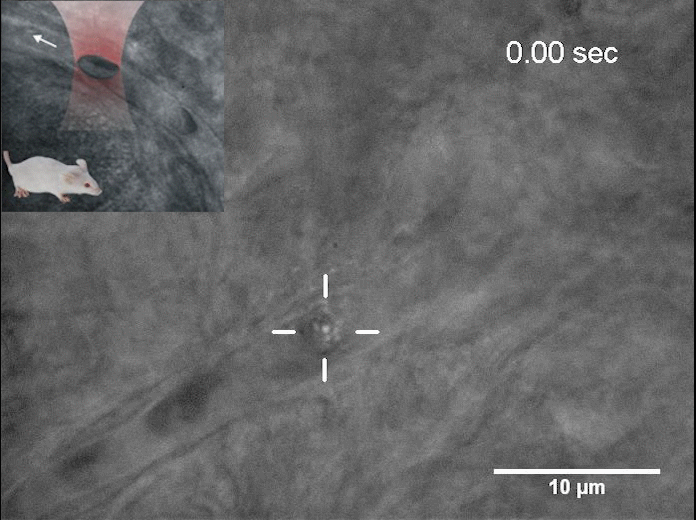
Optical imaging represents a key technology to visualize and understand the structure and activity of cells and biological tissues. Conventional optical systems are designed to be implemented in free space or clean media. However, the presence of optical scattering media scrambles light waves and becomes a key challenge in bio-medical imaging. One particular concept to address this challenge is computational imaging, an imaging modality that exploit physical and mathematical models to computationally reconstruct the image information. Our group is focused on the development of 2-D or 3-D computational imaging methods based on spatial light modulation, for example, single-fiber endoscopy, single-pixel imaging and photoacoustic imaging. Read More
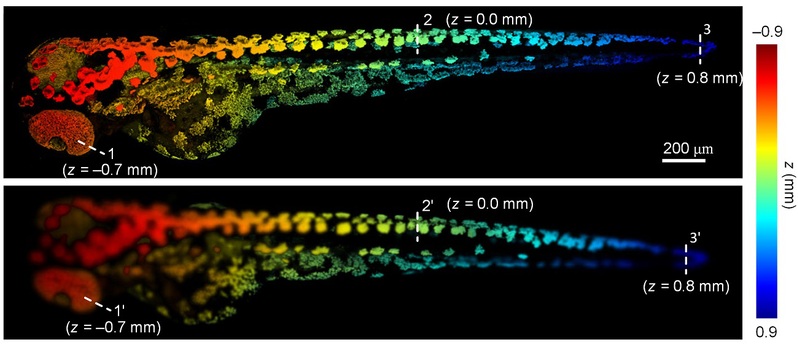
Due to scattering, optical speckles yield when light propagates inside scattering media, for example, biological tissues. Thus, it is challenging to form an optical focus at depths much greater than ~1 mm in soft tissue (the optical diffusion limit). Wavefront shaping aims to overcome the limits of optical diffusion by optimizing the optical wavefront to compensate for phase differences imparted as photons travel along different optical paths in scattering media. In the past decade, various wavefront shaping techniques have been developed to control light propagation in scattering media. In particular, wavefront shaping has the potential to focus at depths of tens of cm in soft tissue, overcoming the limits of optical diffusion. Furthermore, by compensating or exploiting scattering, wavefront shaping holds promise in deep-tissue optical imaging, fiber endoscopy, optogenetics, optical tweezers, and holographic displays. Read More
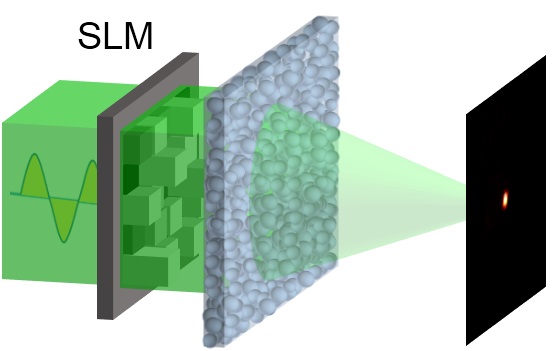
The ability to tailor optical fields is an essential tool in a modern optics and photonics. Structured light refers to the generation, characterization and applications of custom light fields. Shaping arbitrarily complex light fields relies on the control of various light’s degrees of freedom, including amplitude, phase, polarization and coherence. We develop digital techniques based on DMD or SLMs to create non-diffracting light, accelerating light, vector light fields and orbital angular momentum carrying optical vortices. Currently, applications of structured light fields for optical manipulation, imaging, and optical communication are explored. Read More
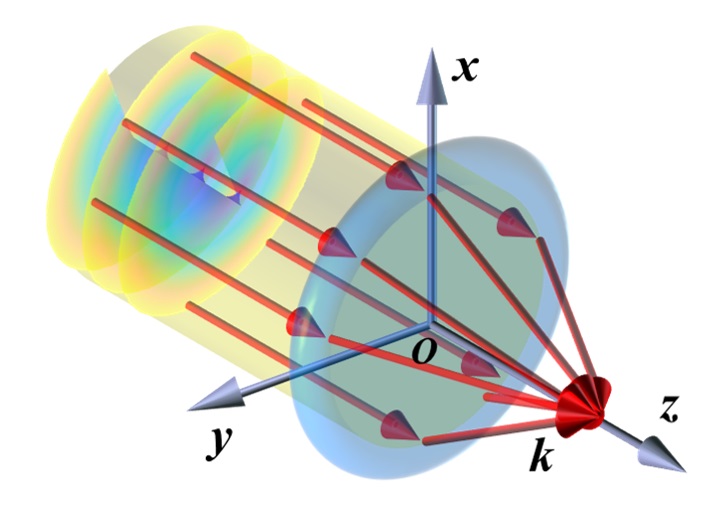